Look At The Protein Below Which Could Be Its Function
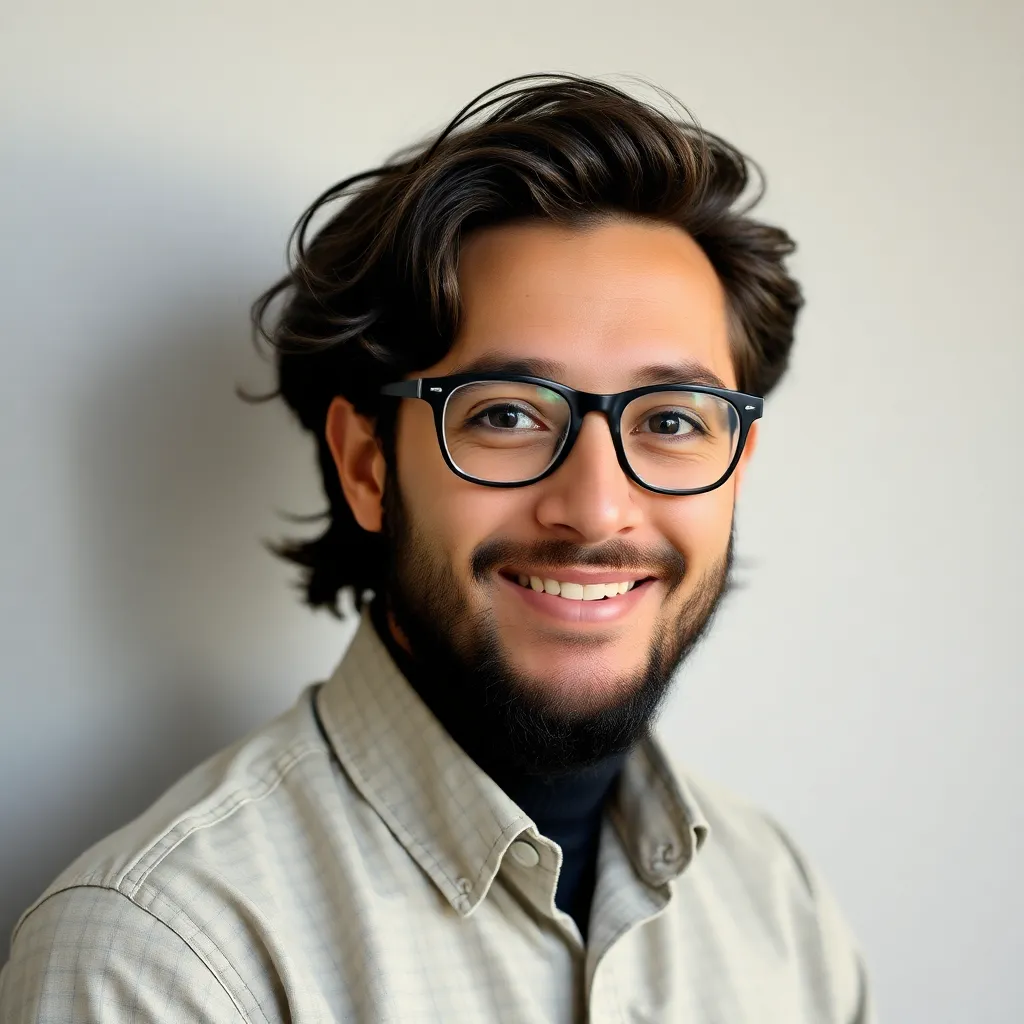
Arias News
May 12, 2025 · 6 min read
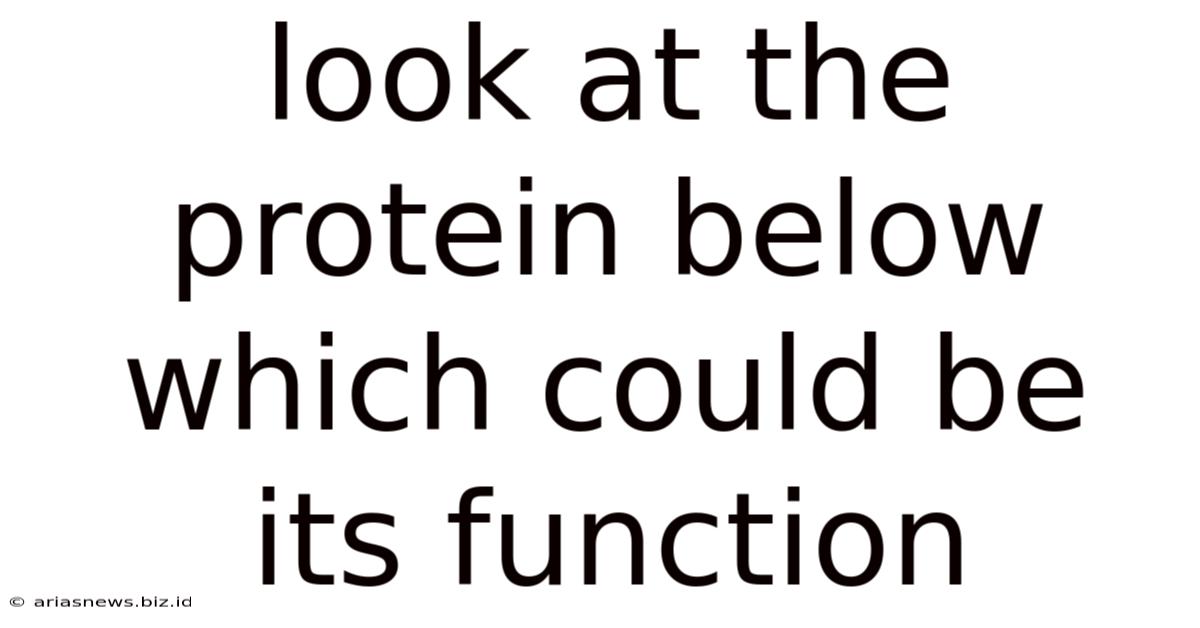
Table of Contents
Deciphering Protein Function: A Deep Dive into Sequence and Structure
Proteins are the workhorses of the cell, carrying out a vast array of functions essential for life. Understanding a protein's function, however, isn't always straightforward. While a crystal-clear image of the protein's 3D structure provides crucial insight, often only a protein sequence is available. This article explores the various methods used to predict and understand protein function, utilizing both sequence information and structural data when available. We’ll delve into bioinformatics tools, evolutionary relationships, and the inherent challenges in this complex field.
From Sequence to Structure: The Foundation of Functional Prediction
The primary sequence of a protein, its amino acid chain, holds the blueprint for its three-dimensional structure and, ultimately, its function. Each amino acid possesses unique chemical properties—hydrophobic, hydrophilic, charged, etc.—that dictate how it interacts with other amino acids and its surrounding environment. These interactions drive the protein to fold into its native conformation, a specific 3D arrangement crucial for its activity.
Predicting Secondary Structure: A First Step
Before even considering the complete tertiary structure, predicting the secondary structure—the local folding patterns like alpha-helices and beta-sheets—provides valuable clues. Algorithms like Chou-Fasman and GOR IV predict secondary structure based on the propensity of individual amino acids to form these elements. While not always perfectly accurate, these predictions offer a starting point for understanding the protein's overall architecture.
Predicting Tertiary Structure: Computational Modeling
Predicting the tertiary structure, the complete 3D arrangement of the polypeptide chain, is significantly more complex. Several computational methods exist, each with its strengths and limitations:
-
Homology Modeling: This method leverages the evolutionary relationship between proteins. If the target protein shows significant sequence similarity to a protein with a known structure (a template), the template's structure can be used as a model, with adjustments made to account for the differences in sequence. This approach is highly reliable when sequence identity is high (above 30%).
-
Ab initio Prediction: This method attempts to predict the structure de novo, without relying on a known template. It employs physics-based calculations to simulate the folding process. While computationally intensive, this approach is crucial for proteins with no homologous structures. Significant advancements have been made in this area, notably with the development of AlphaFold2, which has revolutionized protein structure prediction.
-
Threading: This method involves comparing the target protein's sequence to a library of known protein structures, identifying potential structural templates. It’s less reliant on high sequence similarity compared to homology modeling but still requires significant computational power.
Beyond Structure: Unveiling Function Through Sequence Analysis
Even without a high-resolution structure, the amino acid sequence itself contains considerable information about protein function.
Identifying Conserved Motifs and Domains:
Proteins often contain specific short amino acid sequences, known as motifs, that are highly conserved across different species. These motifs often play a crucial role in the protein's function, such as binding to a particular molecule or catalyzing a specific reaction. Databases like PROSITE and Pfam store vast collections of known motifs and domains. Identifying these conserved regions within a protein's sequence can provide strong hints about its function.
Gene Ontology (GO) Annotation:
Gene Ontology (GO) is a structured controlled vocabulary used to describe the functions of genes and their products. GO terms are hierarchically organized, allowing for a detailed and nuanced description of a protein's role. Many databases annotate proteins with GO terms based on experimental evidence or computational predictions. Analyzing a protein's GO annotations can reveal its involvement in various cellular processes and pathways.
Phylogenetic Analysis: Inferring Function Based on Evolutionary Relationships
Proteins with similar sequences often share a common ancestor and likely perform similar functions. Phylogenetic analysis builds evolutionary trees based on sequence similarities, enabling us to infer a protein's function by comparing it to proteins with known functions in related organisms. This approach is particularly useful when experimental data is limited.
Integrating Experimental Data for Robust Function Prediction
Computational methods provide valuable predictions, but experimental data remains crucial for confirming and refining these predictions.
Biochemical Assays:
Various biochemical assays can directly measure a protein's activity. Enzyme assays measure the rate of enzymatic reactions, while binding assays quantify the affinity of a protein for a specific ligand. These assays provide direct evidence of a protein's function.
Structural Studies:
Techniques such as X-ray crystallography, nuclear magnetic resonance (NMR) spectroscopy, and cryo-electron microscopy (cryo-EM) provide high-resolution structural information. Examining the protein's 3D structure can often reveal active sites, binding pockets, and other functional features.
Genetic Experiments:
Genetic techniques, such as gene knockout or overexpression experiments, can determine a protein's role in a biological system. Observing the phenotypic effects of altering a protein's expression level can provide valuable insights into its function.
The Challenges of Protein Function Prediction
Despite the advancements in bioinformatics and experimental techniques, predicting protein function remains a challenging task.
-
Sequence Similarity Limitations: Homology modeling and phylogenetic analysis rely on sequence similarity. Proteins with low sequence similarity can share the same function, making it difficult to infer function based solely on sequence alone. This is especially true for proteins with convergent evolution.
-
Functional Divergence: Proteins with high sequence similarity can sometimes exhibit diverse functions. This functional divergence occurs through mutations that alter the protein's structure or interactions without significantly affecting its overall sequence.
-
Computational Complexity: Ab initio structure prediction and other complex computational methods require significant computational resources and expertise.
-
Incomplete Databases: The reliability of function prediction depends on the completeness and accuracy of protein sequence and structure databases. Many proteins remain uncharacterized, limiting the scope of comparative analysis.
Case Study: Hypothetical Protein with Unknown Function
Let's consider a hypothetical protein with the following sequence (simplified for illustrative purposes):
MKTILVLLSLAVALALAAALGLGAAAGVAAAVLLLLLAALLLLLLLAVLLLL
Upon initial analysis:
-
Hydrophobicity: The sequence shows a high proportion of hydrophobic amino acids (L, V, I, A). This suggests a potential membrane association.
-
Lack of Conserved Motifs: A search through databases like PROSITE and Pfam does not reveal any significant conserved motifs.
-
Homology Search: A BLAST search reveals limited similarity to known proteins, with low E-values. While this doesn't rule out functional similarity, homology modeling is unlikely to yield a high-quality model.
Therefore, a combination of approaches would be necessary to explore potential functions:
- Secondary structure prediction: Could help identify potential transmembrane helices, supporting the membrane-association hypothesis.
- Ab initio structure prediction: Might provide a 3D model, enabling identification of potential functional sites.
- Experimental validation: Biochemical assays, particularly membrane protein assays, would be essential for confirming its membrane localization and potential function. Further investigations might involve characterizing its interaction with other membrane proteins or lipids.
Conclusion: A Multifaceted Approach to Functional Annotation
Predicting protein function is a complex and evolving field. A robust approach requires integrating multiple lines of evidence, from sequence analysis and structural modeling to experimental validation. While computational methods provide valuable predictions, they should be used in conjunction with experimental data to obtain a comprehensive understanding of a protein's role in biological systems. As technology continues to advance and databases expand, our ability to decipher the functions of proteins will undoubtedly improve, paving the way for new discoveries in biology and medicine. The combination of sophisticated algorithms and experimental verification is key to unlocking the secrets hidden within these fundamental building blocks of life.
Latest Posts
Latest Posts
-
An Education And Career Focused Opportunity That Introduces Students
May 12, 2025
-
Whats The Thickest Layer Of The Earth
May 12, 2025
-
Math Please Excuse My Dear Aunt Sally
May 12, 2025
-
What Fraction Is Equivalent To 1 6
May 12, 2025
-
5 Letter Bird Names Starting With M
May 12, 2025
Related Post
Thank you for visiting our website which covers about Look At The Protein Below Which Could Be Its Function . We hope the information provided has been useful to you. Feel free to contact us if you have any questions or need further assistance. See you next time and don't miss to bookmark.