The Movement Of An Object Around Another Object
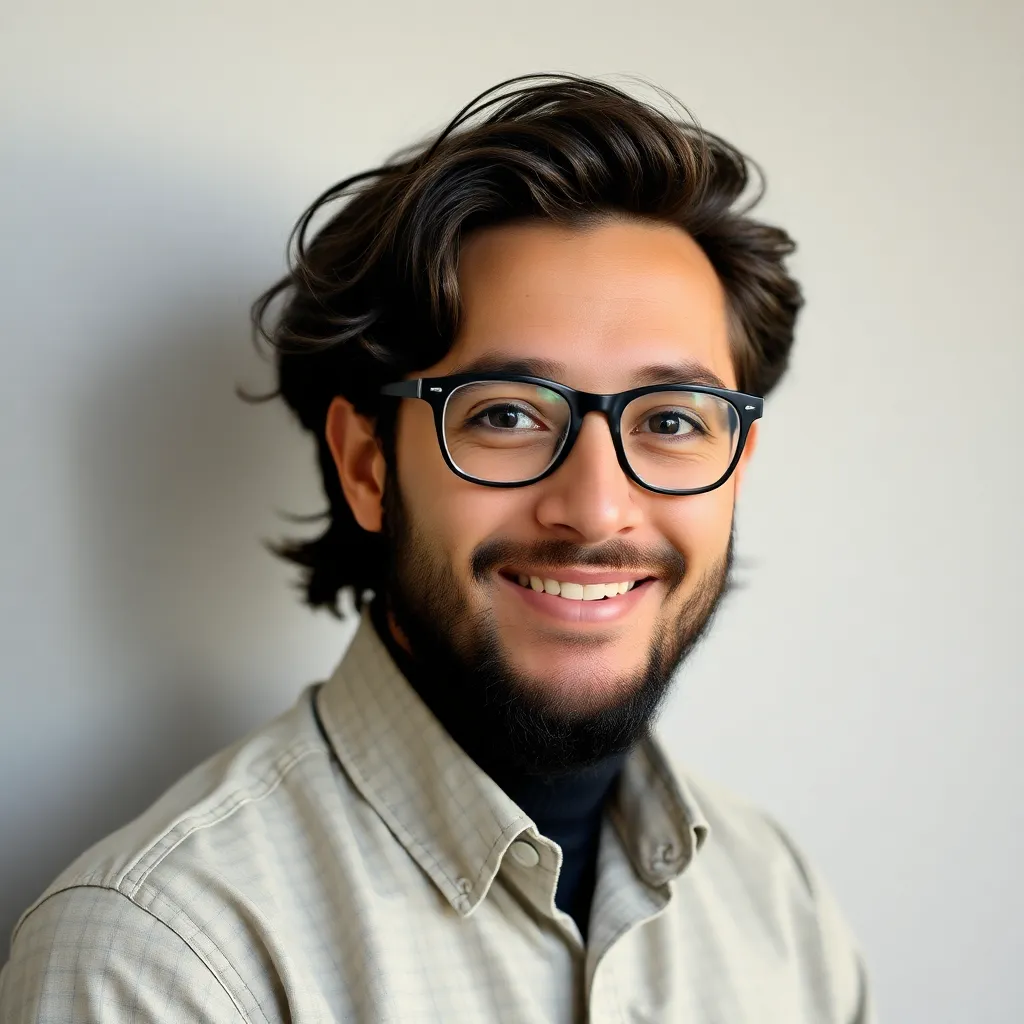
Arias News
May 12, 2025 · 7 min read
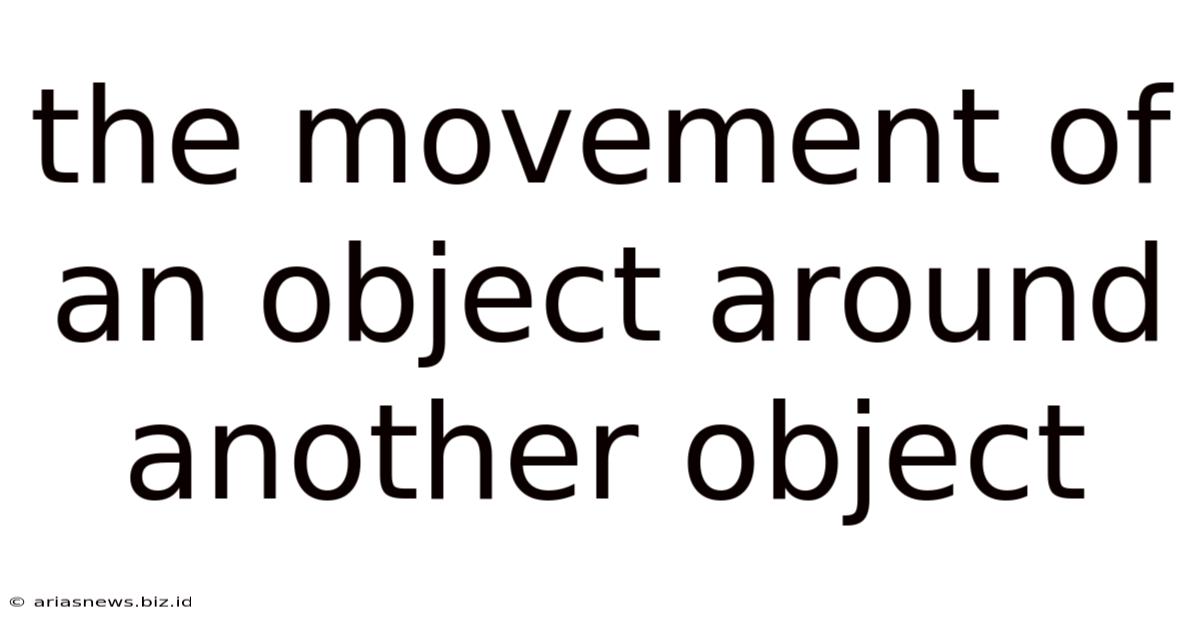
Table of Contents
The Movement of an Object Around Another Object: A Deep Dive into Orbital Mechanics
The movement of one object around another is a fundamental concept in physics, governing everything from the planets orbiting the sun to electrons orbiting an atomic nucleus. This seemingly simple phenomenon is actually a complex interplay of forces and energies, governed by the laws of gravity and, on smaller scales, electromagnetism. This article will explore the intricacies of orbital mechanics, delving into the factors influencing orbital motion, different types of orbits, and the practical applications of this understanding.
Understanding the Fundamentals: Gravity and Kepler's Laws
The primary force driving the movement of one object around another is gravity. Newton's Law of Universal Gravitation states that every particle attracts every other particle in the universe with a force proportional to the product of their masses and inversely proportional to the square of the distance between their centers. This means that the closer two objects are, and the more massive they are, the stronger the gravitational force between them.
This gravitational force is what keeps planets in orbit around the sun, moons around planets, and satellites around Earth. The understanding of these orbital motions was significantly advanced by Johannes Kepler, whose three laws of planetary motion provide a fundamental framework for analyzing orbital mechanics:
Kepler's Three Laws of Planetary Motion:
-
The Law of Ellipses: The path of each planet around the sun is an ellipse with the sun at one focus. This means that the orbit is not a perfect circle, but rather an oval shape. The degree of elongation of the ellipse is described by its eccentricity. A perfectly circular orbit has an eccentricity of 0, while a highly elongated orbit has an eccentricity close to 1.
-
The Law of Equal Areas: A line joining a planet and the sun sweeps out equal areas during equal intervals of time. This means that a planet moves faster when it's closer to the sun and slower when it's farther away. This is a consequence of the conservation of angular momentum.
-
The Law of Harmonies: The square of the orbital period of a planet is directly proportional to the cube of the semi-major axis of its orbit. The semi-major axis is half the longest diameter of the elliptical orbit. This law establishes a mathematical relationship between the size of an orbit and the time it takes to complete one revolution.
Types of Orbits: A Spectrum of Celestial Motion
Orbits aren't all created equal. The characteristics of an orbit are determined by several factors, including the initial velocity, mass of the central body, and the distance from the central body. Different orbital parameters lead to various types of orbits, each with unique properties:
Circular Orbits:
These orbits are characterized by a constant distance between the orbiting object and the central body. They are a special case of elliptical orbits where the eccentricity is zero. While theoretically possible, perfectly circular orbits are rare in nature due to gravitational perturbations from other celestial bodies.
Elliptical Orbits:
The most common type of orbit, elliptical orbits are characterized by their eccentricity, which ranges from 0 (circular) to 1 (parabolic). The orbiting object's speed varies throughout its orbit, being fastest at its closest point (perihelion or periapsis) and slowest at its farthest point (aphelion or apoapsis). Planets in our solar system follow elliptical orbits.
Parabolic Orbits:
These orbits have an eccentricity of exactly 1. Objects in parabolic orbits are only gravitationally bound to the central body for a single pass. After reaching periapsis, they escape the gravitational pull, never to return. Comets sometimes follow parabolic orbits.
Hyperbolic Orbits:
These orbits have an eccentricity greater than 1. Like parabolic orbits, objects in hyperbolic orbits escape the gravitational pull of the central body. However, their velocity is so high that they escape with a significant excess speed. Interstellar objects passing through our solar system often follow hyperbolic orbits.
Geostationary Orbits:
A specific type of circular orbit used for communication satellites. These orbits are synchronized with Earth's rotation, making the satellite appear stationary from a point on Earth's surface. This is achieved by placing the satellite at a specific altitude above the equator.
Geosynchronous Orbits:
Similar to geostationary orbits, but the satellite's orbital period matches Earth's rotation, but it does not necessarily remain above the same point on the equator. The satellite's path traces a figure-eight pattern in the sky.
Factors Influencing Orbital Motion: Beyond Kepler's Laws
While Kepler's laws provide a good approximation of orbital motion, several other factors can influence the path of an orbiting object:
Gravitational Perturbations:
The gravitational pull of other celestial bodies can cause small deviations from Keplerian orbits. These perturbations can lead to changes in the shape, orientation, and size of the orbit over time. For example, the gravitational pull of Jupiter can significantly affect the orbits of asteroids in the asteroid belt.
Atmospheric Drag:
For objects orbiting within a planet's atmosphere, atmospheric drag can cause a gradual decrease in orbital energy, leading to a decaying orbit. This effect is more pronounced at lower altitudes. Satellites in low Earth orbit experience this effect, requiring occasional boosts to maintain their altitude.
Solar Radiation Pressure:
The pressure exerted by sunlight can also slightly affect the orbits of satellites, particularly those with large surface areas and low mass. This effect is subtle but can become significant over extended periods.
Relativistic Effects:
At very high speeds or in very strong gravitational fields, relativistic effects become important. These effects can cause slight deviations from Newtonian predictions of orbital motion. These effects are especially significant for objects orbiting very massive objects such as black holes.
Applications of Orbital Mechanics: From Space Exploration to GPS
The understanding of orbital mechanics is crucial for numerous applications, ranging from space exploration to everyday technologies:
Space Exploration:
Accurate calculation of orbits is essential for planning and executing space missions. Scientists and engineers must carefully calculate the trajectories of spacecraft to reach their destinations efficiently and safely. This involves considering factors such as gravitational perturbations, atmospheric drag, and the fuel requirements for trajectory corrections.
Satellite Communication:
Communication satellites rely on carefully chosen orbits to provide global coverage. Geostationary orbits are particularly important for this application, providing continuous coverage over a specific region. Understanding orbital mechanics ensures that these satellites are placed and maintained in their designated positions.
Global Positioning System (GPS):
GPS technology relies on a network of satellites orbiting Earth. The precise positions of these satellites are constantly monitored and tracked, allowing for accurate determination of location on Earth. The accuracy of GPS depends critically on the accurate understanding and prediction of the satellite orbits.
Astronomy and Astrophysics:
The study of orbits provides valuable insights into the formation and evolution of celestial objects. By observing the orbits of stars in a galaxy, astronomers can determine the mass of the galaxy and infer the presence of dark matter. Similarly, studying the orbits of planets around other stars (exoplanets) can reveal information about their properties and habitability.
Conclusion: A Continuous Journey of Discovery
The movement of an object around another object is a fascinating and complex topic that has captivated scientists and astronomers for centuries. While Kepler's laws provide a foundational understanding, the reality of orbital mechanics is far richer, incorporating the nuances of gravitational perturbations, atmospheric drag, and relativistic effects. The practical applications of orbital mechanics are vast, underpinning crucial technologies and driving advancements in space exploration, communication, and our fundamental understanding of the universe. As our knowledge and technological capabilities continue to evolve, so too will our ability to harness the power of orbital mechanics to explore the cosmos and solve earthly challenges. Further research into areas like chaos theory and perturbation methods continues to refine our models and provide increasingly precise predictions of orbital motion, opening up new possibilities in space exploration and scientific discovery.
Latest Posts
Latest Posts
-
How To Address A Letter To A Nursing Home Resident
May 12, 2025
-
Can Bearded Dragons Eat Brussel Sprout Leaves
May 12, 2025
-
How Many Right Angles Does Trapezoid Have
May 12, 2025
-
Kohler 52 50 02 S Cross Reference
May 12, 2025
-
How Much Is 1 Acre Of Land In Mexico
May 12, 2025
Related Post
Thank you for visiting our website which covers about The Movement Of An Object Around Another Object . We hope the information provided has been useful to you. Feel free to contact us if you have any questions or need further assistance. See you next time and don't miss to bookmark.